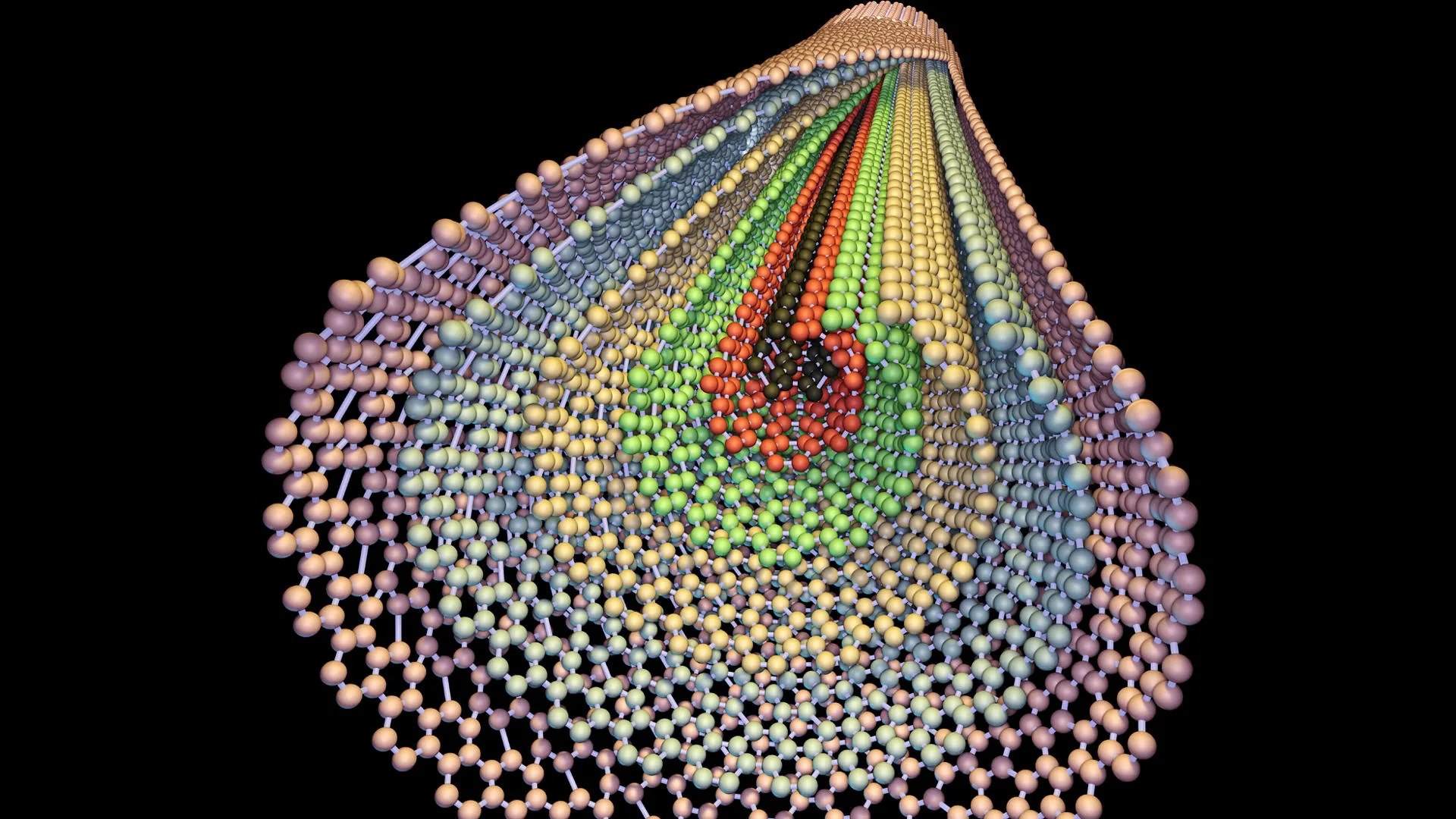
Carbon nanotubes (CNTs) are nanometer-scale structures with immense potential to improve different materials, but inconsistencies in their chemical and electrical properties, purity, cost, and concerns over possible toxicity present ongoing challenges. CNTs are a one-dimensional carbon allotrope made of an sp2 hybridized carbon lattice in a cylindrical shape. Single-walled CNTs are a simple tube, while multi-walled CNTs are nested concentrically or wrapped like a scroll (Figure 1).

These nanoscale materials feature a high Young’s modulus and tensile strength and can have either metallic or semiconducting electrical properties. Controlling their atomic arrangement (chirality) affects their conductivity, and because of this, researchers have been trying to understand how synthesis parameters can be used to generate CNTs with predictable electrical properties. The development of various chemical vapor deposition (CVD)-based recipes within the last 20 years to synthesize CNTs has improved this situation.
As we’ve seen in our analysis of the CAS Content Collection™, the world’s largest human-curated collection of published scientific information, the increase in patent activity indicates a high amount of interest in commercial applications for CNTs (Figure 2).

Patent growth in battery technologies
The strength and conductivity of CNTs make them useful in battery materials where they provide mechanical support during charging and discharging cycles, thereby extending battery life, while also acting as electrically conductive pathways. Our analysis of CNT-related publications revealed that patent publications reference batteries about three times more often than journal publications. (Figure 3)
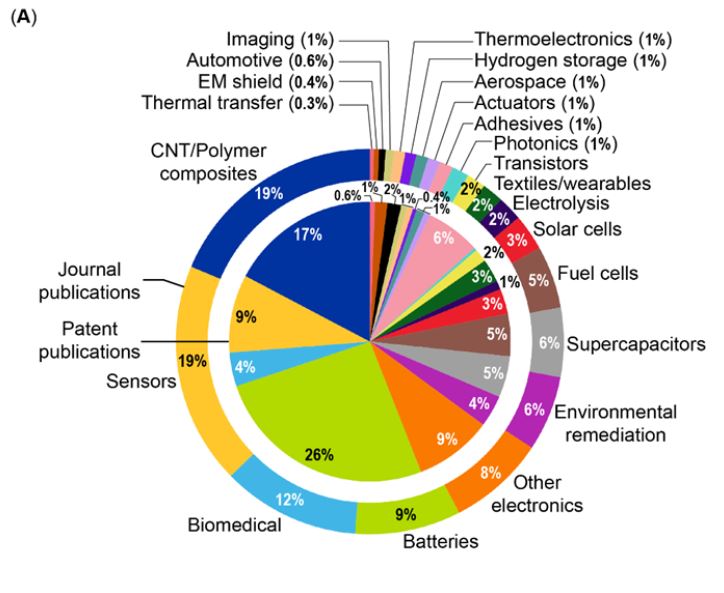
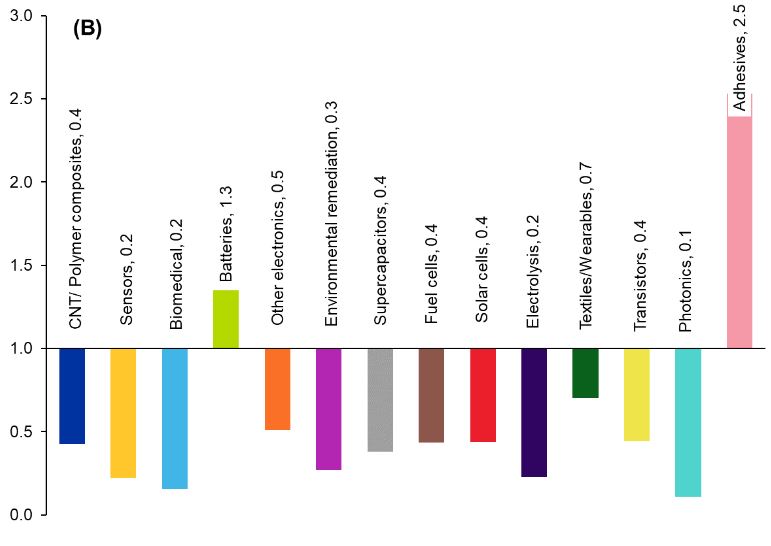
These publication trends reflect the commercial viability of CNTs, and their potential to be used in a growing number of battery applications for EVs and smart devices. Numerous battery patents come from China, Korea, and the U.S., likely driven by large consumer electronic industries and electric vehicle manufacturers in these regions.

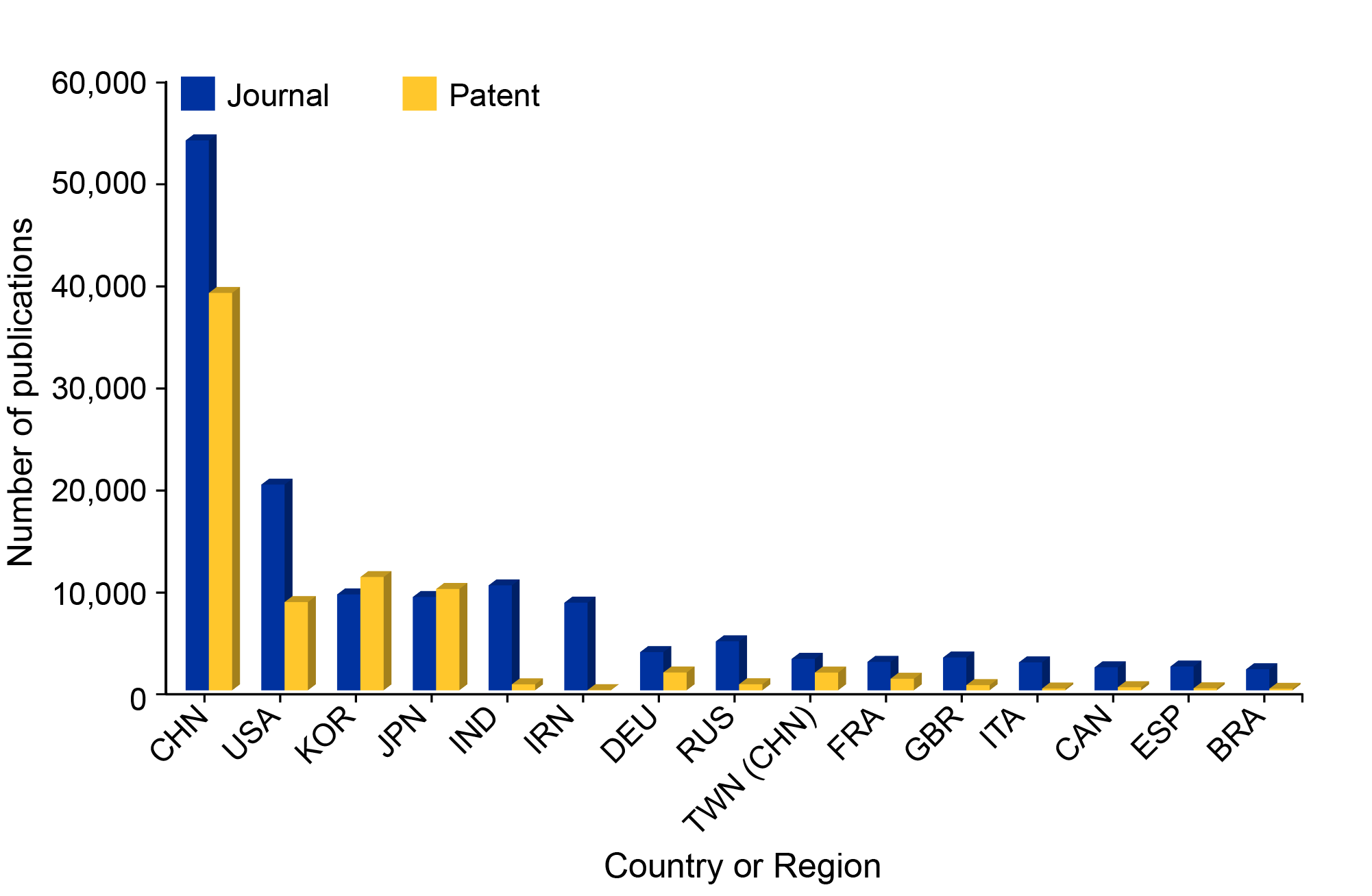
CNT publications comprise several battery chemistries — lithium-ion batteries dominate, but publications involving zinc-ion batteries have shown a notably high growth rate in recent years. In zinc-ion batteries, the role of CNTs is to form high-surface-area, mechanically resilient, flexible, and electron-conductive scaffolds that support other active cathode materials. This improves rate capability and capacity retention after repeated cycling.

The emergence of MXene nanomaterials is also spurring interest in their use in battery electrodes. In this application, CNTs can be used to control the spacing between MXene sheets and prevent them from re-stacking, which improves ion transport. CNT-MXene combinations can also be used in supercapacitors for energy storage applications, although these publication citations are primarily in journals, suggesting they are not ready for large-scale commercialization.
Steady growth in composite applications
Since their discovery in the early 1990s, the primary applications of CNTs have been in composites, where they contribute mechanical strength and high thermal and electrical conductivity. They are found in products ranging from sporting goods to industrial coatings. We’re now seeing CNT publications cite their usage in hydrogels, epoxy resins, and polymers, including the renewable and biodegradable polymer poly(lactic acid) (PLA). In some of these cases, CNTs are used to reinforce brittle materials and help them withstand mechanically demanding applications. (Figure 6)

Other notable citations involve CNTs combined with polymer matrices for electromagnetic shielding. The large amount of both journal and patent publications relating to composites demonstrates the viability of CNT composite innovations in commercial and more experimental applications.
Research interest in sensors and biomedical uses
Two fields where CNT research has not reached large-scale commercialization but has significant potential are sensors and biomedical uses such as drug delivery. Sensors constitute the second-largest application referenced in CNT-related journal publications. CNTs can be used to enable both chemical sensors and mechanical sensors, such as those used to measure strain or pressure for human motion detection. Their strength, aspect ratio, conductivity, and ability to be chemically functionalized make them useful in converting and transmitting these stimuli, which explains their potential in these devices.
Our analysis revealed that, while 14% of CNT journal publications relate to sensors, only 7% of patent publications do. It’s possible that at this stage of their development, the fabrication of some sensors on a laboratory scale requires more labor or expensive materials to assemble than would be commercially viable. Alternatively, laboratory-conceived sensors may be proofs-of-concept or utilize target stimuli that are of relatively lower commercial interest for future development.
Likewise, biomedical applications of CNTs make up 9% of journal publications but only 3% of patent publications. CNTs have been used as a reinforcing material for drug-delivery stents, a conductive material on nerve catheters, and an antibacterial and structural material for bone implants. These applications are promising, but CNTs can exhibit toxicity depending on their purity, size, and shape. Because of this, more clinical trials are needed to fully explore their specific biomedical uses prior to commercialization.
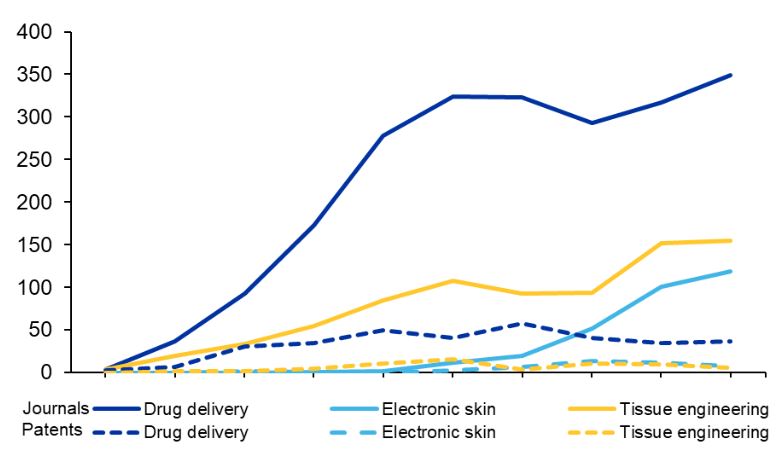
Potential for environmental remediation applications
CNTs’ high surface area and chemical customizability make them ideal for absorptive and other types of environmental pollutant removal. They can also be modified and tailored using co-catalysts to enhance reactivity with certain pollutants. For example, a lanthanum-gadolinium-iron oxide-perovskite reinforced with CNTs showed improved photocatalysis of the degradation of phenol red compared to a CNT-free perovskite.
CNTs can also be used as adsorbents for wastewater treatment. They have successfully removed ciprofloxacin, a difficult-to-remove antibiotic that is harmful to aquatic organisms. We’ve since seen significant growth in citations comparing the performance of CNTs and biochar for removing environmental pollutants.
Journal articles relating to CNTs and environmental remediation are much more numerous than patent publications. Possible explanations for this are that their cost makes them economically infeasible for remediation activities, or that more basic research is needed to improve their efficiency.
CNT challenges to overcome
The applications of CNTs span fields as diverse as energy storage, durable goods, electronics, environmental remediation, and biomedical devices. Their unique properties make them valuable additions to a wide range of products, but the need for precision in these applications complicates their usage. Our analysis indicated that tuning synthesis parameters to control size and chirality remains an active area of research. Because chirality determines the electrical properties of CNTs, this research may lead to methods that can overcome some of these challenges.
Additional research, particularly in vivo and clinical trials, will help scientists better understand toxicity risks in biomedical applications. The more that CNTs are generated, the more researchers will understand and control their purity. Scaling up production can also address current cost issues, which could spur the further development of CNTs for environmental remediation solutions and more cost-sensitive applications.
Significant research hurdles remain, but with continued innovation, CNTs can achieve their extensive commercial potential.