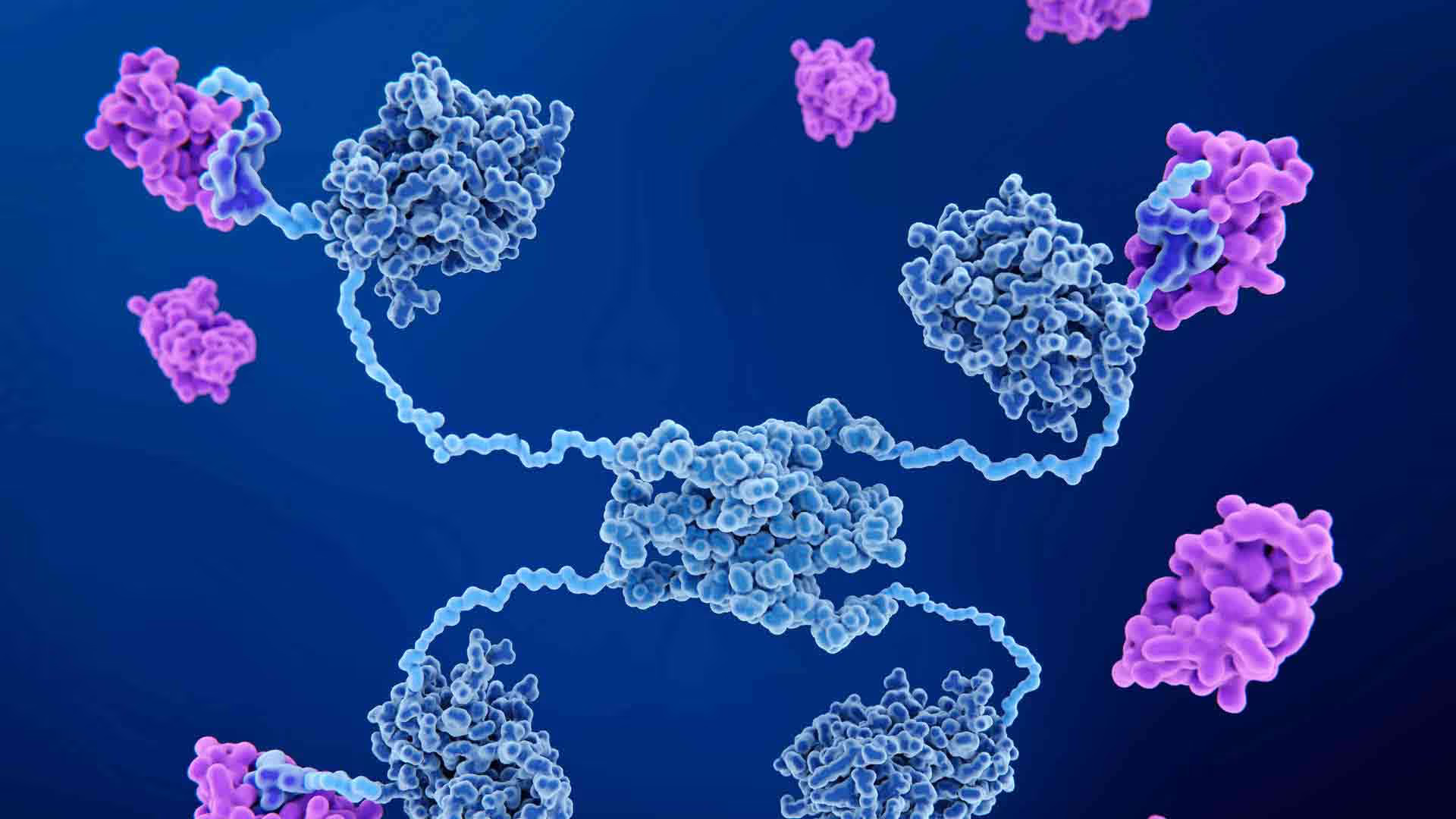
Many of today’s medications target proteins, acting on an individual protein or enzyme. Researchers have identified about 20,000 such proteins that drugs can target. There are, however, vastly more protein-protein interactions (PPIs) that play a critical role in diseases, and some drugs are now targeting these interactions to inhibit them from taking place. PPIs are crucial to many types of cellular processes, and when these processes go wrong, whether through mutations or modifications, they can be a primary factor in the development of cancer, infectious diseases, and neurodegenerative diseases.
It’s estimated that over 650,000 PPIs could be targeted with medications. Until recently, inhibiting PPIs with small molecule drugs proved challenging. This is because the interface of proteins in a PPI is large and was believed to be a difficult environment for small molecules to inhibit the association of these much larger proteins.
Typically, PPIs have been targeted not with small molecule drugs but with antibodies and peptides, several of which have been approved for clinical usage including Opdivo and Keytruda. These forms of PPIs are highly specific to their targets, but can have issues with immune system reactions and, in the case of peptides, poor solubility and stability.
Recent breakthroughs in understanding the structure and function of proteins involved in PPIs are changing how researchers approach small molecule drug development for these targets. Advancement of drugs to late-stage clinical trials means new therapeutics (especially for cancers) may be near.
New findings open possibilities for small molecule drugs to inhibit PPIs
There are two ways a PPI inhibitor works. The first, known as orthosteric inhibition and the primary mode of action, is where a small molecule binds at the interface of two proteins, causing a competitive inhibition of the second protein’s binding. The second way, allosteric, is where a small molecule binds to a site outside the protein-protein binding site interface and causes a contortion of the protein. This results in an alteration of the binding interface, thereby inhibiting the interaction (see Figure 1).
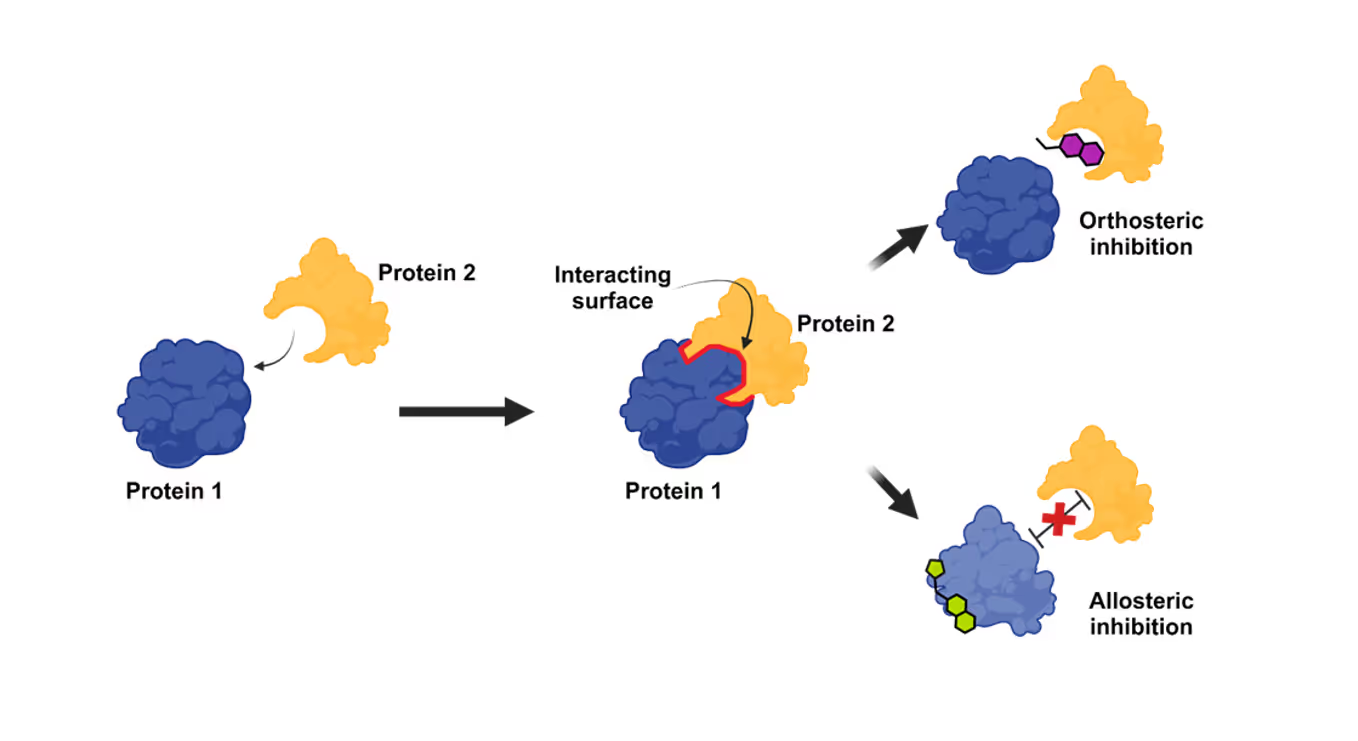
Previously, researchers believed that small molecules would struggle to bind to PPI interfaces because these tend to be large and flat. However, researchers recently determined that certain collections of amino acid residues in the binding interface, or “hot spots,” can be found where ligands can interact to inhibit the PPI. They have also found that as some potential therapeutics bind, they induce the development of a binding pocket by changing the tertiary structure of the PPI interface.
These findings, along with the initial U.S. FDA approval of the small molecule PPI inhibitor Venetoclax in 2016, have encouraged the development of many more PPI inhibitors that are showing promise in treating blood cancers and solid tumors. We’ve noted an increase in publications relating to PPI inhibitors in the CAS Content CollectionTM, the largest human-curated repository of scientific information. This growth is most noticeable after 2018, which demonstrates an accelerated interest within the past few years (see Figure 2).

Advancement in clinical trials brings PPI inhibitors closer to market for cancer treatment
Although Venetoclax is being evaluated against multiple types of cancer, it was originally approved for chronic lymphocytic leukemia. It’s a selective Bcl-2 (B-cell leukemia/lymphoma 2 protein) antagonist that controls the mitochondrial apoptotic pathway. Its dysregulation in cancer can lead to a check on apoptosis (programmed cell death) and enhance tumor survival and growth. Conversely, inhibition of the protein leads to the activation of apoptosis and attacks on cancer cells.
The success of venetoclax has led to Bcl-2 being one of the most common targets for PPI inhibitors. Lisaftoclax, developed by the Ascentage Pharma Group, is now in Phase III clinical trials targeting chronic lymphocytic leukemia like venetoclax. The blood cancer myelofibrosis is also the target of a new PPI inhibitor in Phase III. This innovation, pelabresib, a compound from Constellation Pharmaceuticals, inhibits the interaction of BET (bromodomain and extra-terminal domain) proteins, which serve as transcriptional co-regulators through the recognition of the acetylated histone tails associated with certain genes.
Advances in other PPI inhibitors may soon treat solid cancers as well. For example, small molecules targeting the MDM2/p53 interaction have reached Phase III clinical trials. The p53 protein can halt cell division and induce apoptosis, both of which can prevent cancer cells from spreading. MDM2 is a negative regulator of p53, so preventing this interaction allows p53 to exert its anti-cancer effects (see Figure 3). The drug navtemadline by Kartos Therapeutics targets this PPI and is in Phase III for treating endometrial cancer.
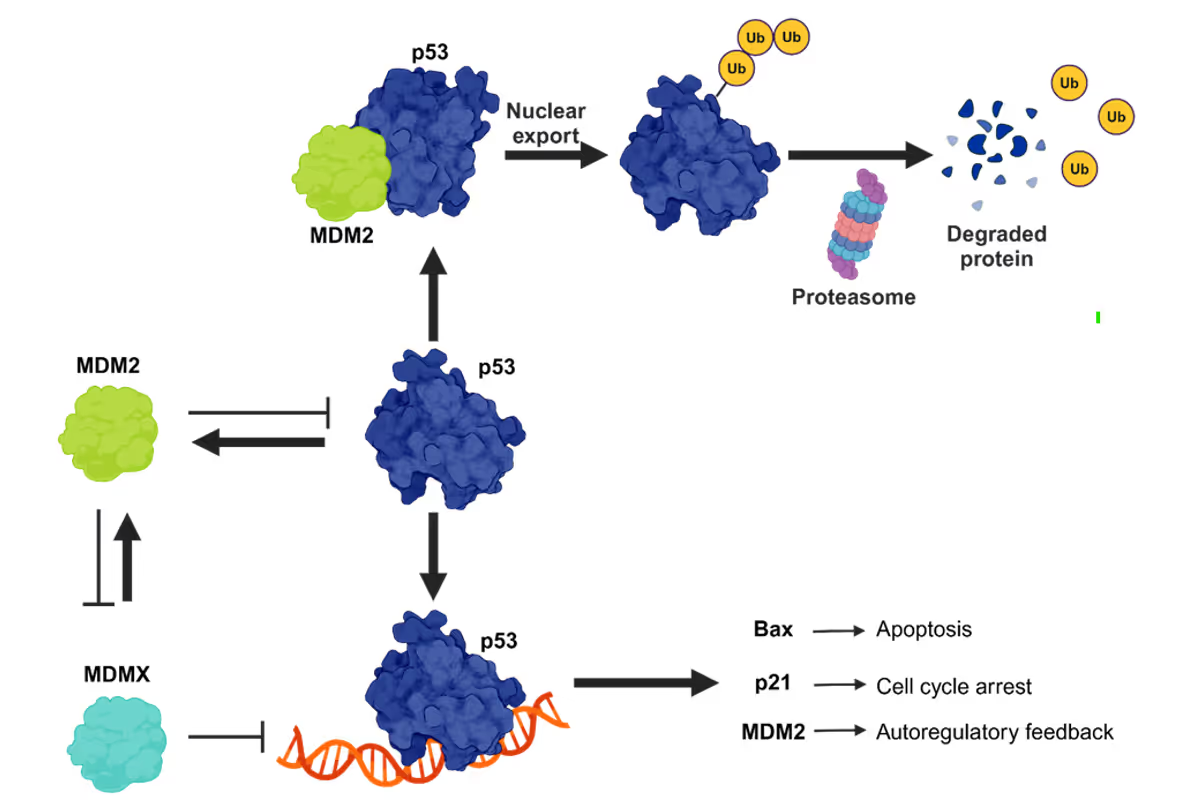
The target XITP (x-linked inhibitor of apoptosis proteins) is similar to MDM2 by inhibiting other proteins from carrying out apoptosis. By stopping this protein from interacting with the pro-apoptic protein caspase-9, caspase-9 can stop the growth of cancer cells. The drug xevinapant, which targets this PPI, is now in two separate Phase III clinical trials to treat head and neck cancers (see Figure 4).

A number of Phase II trials are also in progress, targeting different PPIs to treat blood cancers and solid cancers. These efforts reinforce how promising the MDM2/p53 pathway is for new cancer treatments:
- Pelcitoclax: Targets the Bcl-2 family of proteins, studied for treating small-cell lung cancer and other solid tumors.
- Ziftomenib: Targets the Menin-MLL protein to treat mixed lineage leukemia.
- APG-115: Targets the MDM2/p53 interaction for treatment of solid tumors.
- Idasanutlin: Targets the MDM2/p53 interaction for treatment of acute myeloid leukemia.
- Siremadlin: Targets the MDM2/p53 interaction for treating soft tissue carcinoma.
AI assists in overcoming research challenges
PPI inhibitors as therapeutic treatments are successful, but the sheer number of PPIs in the human body — as many as 650,000 — as well as their size, makes it challenging to characterize all their structures. Understanding the structure of the protein/ligand complex is key to developing small molecule drugs to treat these protein interactions. However, growing high-quality crystal structures of proteins is costly, time-consuming, and difficult for proteins the size of those involved in PPIs.
AI-driven modeling programs, such as AlphaFold, can generate predictive protein structures for nearly every human protein. This is unlocking a greater understanding of protein structures, their binding interfaces, and, thus, potential compounds to inhibit interactions between such proteins. These new technological tools are providing researchers with much more information on PPI complexes, hot spots, and binding pockets. Underscoring the importance of this technology is that the scientists responsible for advances in predictive protein modelling, David Baker, Demis Hassabis, and John Jumper, received the 2024 Nobel Prize in Chemistry.
The field of PPI inhibitors is still young, and there is much work to be done to better design effective small molecule therapies. Antibodies and peptides remain as options for drug development, but challenges with these treatments such as immunogenicity and poor solubility make it vital that small molecule drugs are still developed.
As the growth in clinical trials shows, researchers are building on recent breakthroughs quickly, and doctors may soon have new tools in their arsenal to improve cancer treatment.