2024 saw many notable scientific advancements, and progress shows no sign of slowing down. At CAS, we’re constantly keeping our finger on the latest research, and we’ve identified eight key trends that could drive major scientific breakthroughs in 2025. Recent developments in these areas span diverse subjects like AI in research, precision medicine, and new battery technology. Together, they hold the possibility of improving our health, our environment, and the opportunities for businesses and entrepreneurs to better leverage new research and technology.
You can also watch the recording of our expert panel from CAS, the University of California, Berkeley, Oak Ridge National Laboratory, and POLARISqb discuss these trends and related areas they’re most excited to be involved with in 2025.
CRISPR therapeutics pipeline gaining momentum
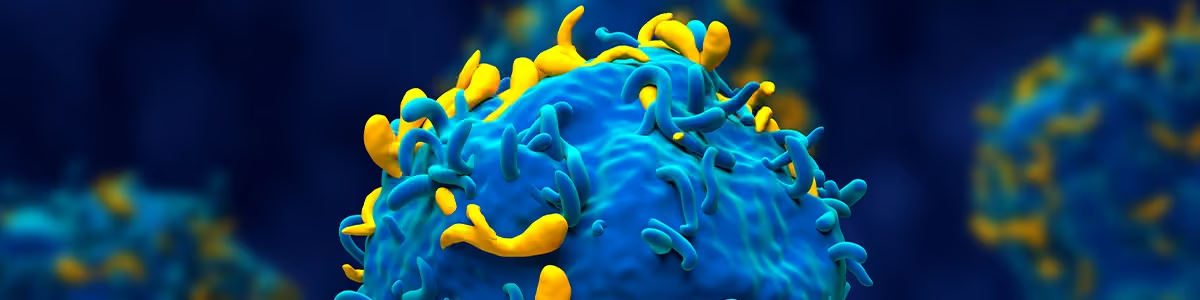
Cutting-edge gene editing technologies, particularly CRISPR, are revolutionizing the landscape of drug discovery. Casgevy was the first therapy to be approved by the U.S. FDA that was developed using CRISPR-Cas9 gene-editing technology, and many new CRISPR-based therapies targeting a broad range of diseases have entered drug discovery pipelines and trials since.
The rapid development of base editing, prime editing, and even CRISPR-based epigenetic modulation has propelled CRISPR to the forefront of drug discovery, with potential applications in oncology, genetic disorders, viral infections, and autoimmune diseases. Correcting mutations, silencing harmful genes, or introducing protective changes in cells marks a paradigm shift from symptom management to therapies with curative potential for patients.
A few examples of how CRISPR is enhancing therapeutic approaches include:
- Knocking out genes that inhibit T-cell function or enhance their ability to target cancer cells, leading to more potent and less toxic CAR-T therapies.
- Adding controllable safety switches that can stop and reverse CAR-T cell therapies based on individual genetic responses.
- Identifying genes and proteins in cancer cells, revealing new targets for PROTACs.
On its own, CRISPR’s versatility as a gene-editing tool allows for gene correction and silencing, which holds potential for curative treatments for monogenic diseases and viral infections. However, it’s the complementary nature of these technologies—CRISPR, CAR-T, and PROTACs—that is most exciting, enabling collaborative drug discovery across multiple technologies. New therapies that rely on CRISPR’s flexibility can address previously elusive aspects of disease biology and patient needs, shaping a future where combination approaches will yield more effective therapies.
Solid state batteries innovation boosting lithium advances
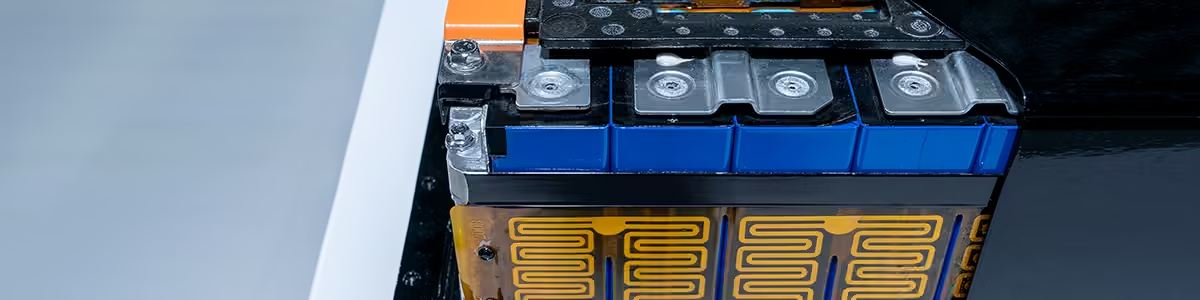
Lithium-ion batteries (LIBs) are ubiquitous in today’s EVs and many consumer electronics. As a result, new research is focused on developing the next generation of LIBs better suited to these applications.
Solid-state batteries are one of the emerging technologies gaining momentum because they have the potential to address many of the critical issues hindering EV adoption. What are the advantages of solid electrolytes over the liquid or gel electrolytes commonly used in today’s LIBs?
- Safer and less prone to fires
- More durable and able to be discharged many more times
- More compact, packing more energy into the same volume and weight
- Faster to recharge
- Resistant to cold weather performance degradation
Despite these benefits, some industry experts remain skeptical, noting that this technology still faces cost, manufacturing, and production validation hurdles as it is tested in real-world conditions.
Overall, auto manufacturers are optimistic, as evidenced by the widespread growth of industry investments in this technology:
- Honda has recently unveiled an all-solid-state EV battery production line, estimating that these batteries will be 50% smaller.
- SAIC, one of the biggest Chinese automakers, has said it will start mass production of second-gen solid-state batteries in 2026.
- Nissan has also announced plans to launch an EV with solid-state batteries by 2028.
Given all this investment, it appears the tipping point for solid-state batteries may be on the horizon in the years to come. Beyond vehicle applications, battery technology is being carefully watched in many industries, as each new breakthrough enables new applications and possibilities.
Data-quality emerging as key driver for AI success

AI will continue to dominate headlines in 2025, but as these technologies are integrated into numerous industries, the discussions on optimizing AI outcomes are shifting from algorithms to data.
Data is the foundational fuel used to train and inform all machine learning applications. Large language models (LLMs) such as ChatGPT continue to show significant limitations when used for specialized scientific and technical applications. This is in part because these tools are limited in their ability to process chemical structures, tabular data, knowledge graphs, time series, and other forms of non-text information.
The importance of data quality and diversity to AI outcomes has been well studied. However, fit-for-purpose data is often not readily available for specific projects. To improve AI for research across a wide array of scientific applications, researchers are demanding better quality and more specialized data sets targeted to the intended model application. This has already bolstered drug repurposing research and facilitated computer-aided drug design. Approaches used to close the data quality gap and improve outcomes for AI applications include:
- The development of customized datasets to train AI, such as the one being used by MIT and Toyota to train self-driving vehicles.
- Compound AI systems that leverage more data sources and reduce “hallucinations” and inaccurate results.
- A “mixture of experts” approach that trains multiple smaller sub-models on specific tasks rather than using one large model.
- Use of synthetic data generated by other AI models to train new models when sufficient real-world data is unavailable.
Materials science innovation supporting progress on climate action
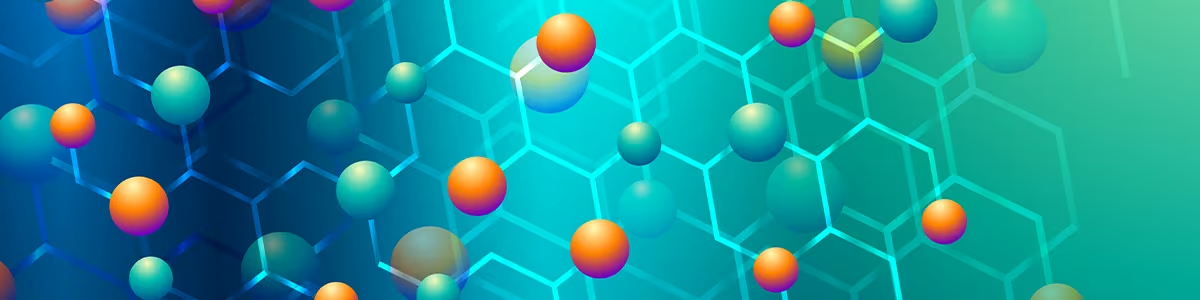
Under the Paris Agreement, many countries have committed to significantly reducing greenhouse gas emissions by 2030 with the goal of reaching net zero by 2050. An array of scientific innovations is enabling progress toward that goal, including new materials science technologies that offer fresh ways to address key challenges.
Metal-Organic Frameworks (MOFs) are highly porous crystalline materials composed of molecular cages formed from metal ions coordinated to organic molecules. These materials exhibit an extensive diversity of properties such as high surface areas, tunable pore sizes, and flexibility to pressure and temperature changes, making them suitable candidates for gas storage, gas separation, magnetism, catalysis, and electrical and optical applications. BASF is pioneering production of MOFs at commercial scale for carbon capture due to their exceptional surface area and tunable properties, and other companies are not far behind.
As the climate warms, energy-efficient air conditioning will also be critical, and MOF-based coatings are also proving useful for that application. When coated on AC parts, MOFs have efficiently extracted humidity from the passing air, reducing the cooling energy required by up to 40%.
Covalent organic frameworks (COFs) are also showing great potential in energy storage, catalysis, and gas separation. Unlike metal-organic frameworks, COFs are completely organic. These two- or three-dimensional structures also have higher thermal and chemical stability compared to MOFs, and recent research shows that this stability enables these materials to operate continuously and cleanse the atmosphere. COFs have also proven effective in pollution control applications such as detecting and removing perfluorinated compounds from drinking water.
Materials science research is expected to play an increasingly important role in sustainability initiatives over the coming years through the development of new materials with lower environmental impact. We expect this to result in broader innovation in renewable technologies and processes that mitigate the effects of pollution, improve energy efficiency, and impact many other sustainability goals.
Molecular editing boosting innovation in drug discovery

Traditionally, chemists have relied on a large but bounded set of known reactions to synthesize complex organic molecules. However, emerging synthetic approaches are making new molecular scaffolds and shapes more accessible, potentially catalyzing an exciting new wave of innovation in organic and medicinal chemistry.
Molecular editing is a technique that allows for precise modification of a molecule’s structure by inserting, deleting, or exchanging atoms within its core scaffold. Unlike the traditional approach of building up new large molecules by assembling smaller parts through a series of stepwise reactions, molecular editing enables chemists to create new molecules by precisely modifying existing large molecules. This empowers them to create new compounds more efficiently and cost-effectively, and by reducing the total synthetic steps, decrease the volume of toxic solvents and energy requirements for many transformations.
Potentially the most compelling aspect of molecular editing is its anticipated positive impact on innovation. Causes and remedies for a perceived “innovation crisis” in the pharma industry have been debated over the past decade. However, there is little question that multiplying the paths chemists have at their disposal to reach a desired structure is key to increasing the volume and diversity of molecular frameworks being considered for drug candidates, fertilizers, materials, and many other applications.
In combination with emerging AI-based synthetic applications that are already helping chemists identify and prioritize synthetic pathways, these new synthetic approaches could drive a multi-fold increase in chemical innovation over the next decade.
Waste management innovations advancing the circular economy
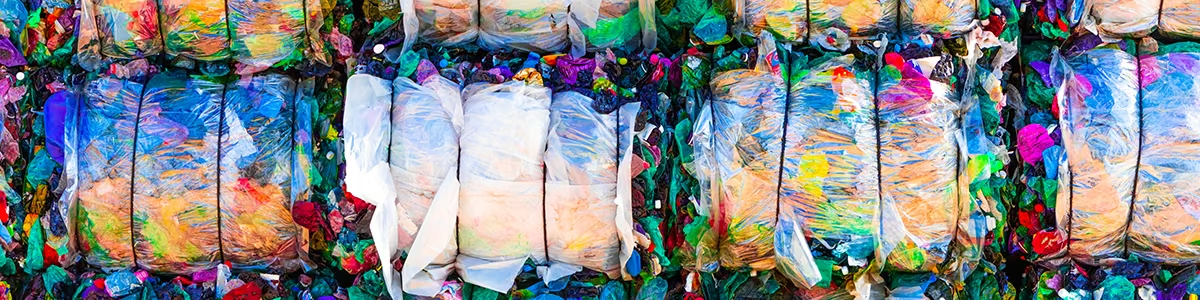
The UN Global Waste Management Outlook for 2024 estimates that, without radical change, the combined annual costs of waste management—including the hidden costs of pollution, poor health, and climate change—will double by 2050. As such, new technologies are advancing progress within a circular economy where reuse and recycling take on larger roles:
- Besides traditional recycling methods like pyrometallurgy and hydrometallurgy, new battery recycling methods are being developed that reuse valuable metals like lithium, cobalt, nickel, aluminum, iron, and manganese with methods such as bioleaching, direct recycling, and electro-hydrometallurgical processes. These new approaches not only keep dangerous chemicals from entering the environment, but also recapture valuable, often scarce elements used in many popular technologies.
- Biomass conversion technologies such as hydrothermal carbonization are being used to convert waste-to-energy, turning wet biomass, organic waste, and agricultural residues into hydrochar, a carbon-rich material used in electricity generation and soil conditioning, and biochar, a material used for soil improvement.
- Plastic-eating bacteria are improving the efficiency of plastic recycling by regenerating monomers from waste—spurred on by the discovery of Ideonella sakaiensis 201-F6, a bacterium with the enzymes IsPETase and IsMHETase that break down polyethylene terephthalate (PET) into its two environmentally benign monomers, ethylene glycol and terephthalic acid. If this technology can be scaled, it will help keep up with the world’s ongoing “addiction to plastic.”
New, innovative efforts like these don’t just offset the costs of waste disposal but provide better financial incentives as new technologies come online. Keep an eye on technologies that can recover or create new valuable resources from waste as manufacturers, energy producers, governments, and waste management companies invest in innovation aimed at making waste management less expensive to enhance sustainability and improve the profitability of manufacturing processes.
Quantum computing getting practical
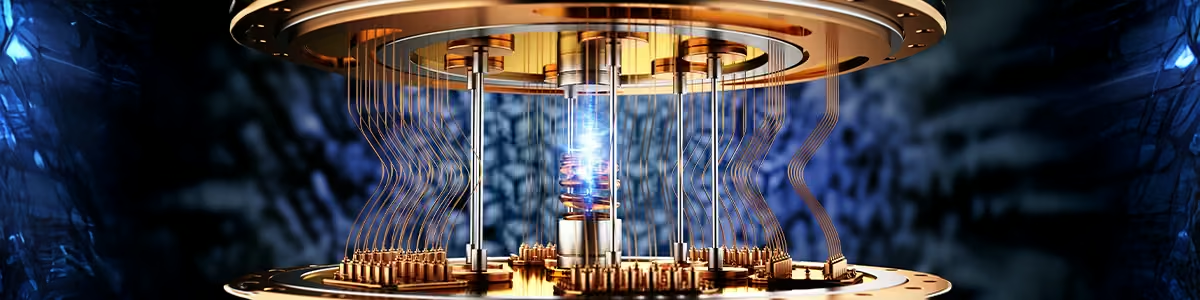
The United Nations has proclaimed 2025 as the International Year of Quantum Science and Technology (IYQ). While quantum computing technology isn’t yet widely commercialized, it’s making steady progress toward real-world application in scientific R&D. For example, Cleveland Clinic and IBM recently installed the world’s first quantum computer dedicated to healthcare research and are beginning to apply its capabilities to tackle drug discovery questions that even modern supercomputers could not answer. Researchers are exploring how quantum computing will accelerate drug discovery by enabling more complex simulations of molecule behaviors and efficient modeling of protein folding. This creates an opportunity for quantum computing to drive significant progress in a short period of time as implementation ramps up.
Beyond drug development, quantum computing could solve complex challenges in many other fields. For example, agriculture researchers are testing applications in fertilizer calculations and field monitoring that could optimize crop yields to enhance food production while minimizing environmental damage. Quantum computing is also anticipated to enable more accurate weather forecasting by identifying patterns within the large volume of global data and more quickly evaluate multiple disparate scenarios generated by different models.
Google’s recent introduction of its new Willow chip, as well as an announcement by Microsoft and Atom Computing of their intent to deliver commercially available quantum computers in 2025, show how rapidly this technology is advancing. While we don’t anticipate it will dominate the technology landscape in the next few years, as there are still many challenges to scaling this technology, quantum computing is emerging as a key driver in several fields.
Omniomics: The next single-cell revolution

Investment in new single-cell analysis technologies has exploded in recent years, and these techniques are now being applied to advance critical progress in early disease detection, prenatal screening tests, biomarker testing, liquid biopsies, and biologic drug development. The market for single-cell analysis technologies related to genomics, transcriptomics, and proteomics was estimated at USD 4.34 billion in 2023 and is projected to grow at a CAGR of 18.7% from 2024 to 2030.
The next frontier ramping up in single-cell research is multi-omics. By combining multiple single-cell techniques, multi-omic approaches provide a more complete picture for researchers and clinicians:
- In terms of drug discovery, multi-omics can assist in the design of therapeutics and advance vaccine development as simultaneous integration of various single-modality omics methods at the single-cell level can help us understand various biological processes, pathways, and disease mechanisms.
- Multi-omics is also being used to establish cellular linkage trees, which make it possible for cancer researchers to study the impacts of epigenetic effects and genetic mutations simultaneously at the single-cell level.
- In glioblastoma patient models, multi-omics has shown potential to improve understanding of intratumor heterogenicity, thereby informing patient-specific therapies.
Beyond multi-omics, omniomics strives to merge all omics data to give us an integrated picture of human biology at the cellular level, uncovering complex biological interactions and disease mechanisms with greater accuracy. This approach has widespread applications for drug innovation and could accelerate the development of targeted therapies. It also has profound implications for improving precision medicine, providing a deeper understanding of different cells within a tumor and how they change over time, thus paving the way for personalized therapeutic strategies.