
"We are in the midst of a therapeutic revolution," according to the authors of a recent review article in Frontiers in Bioengineering and Biotechnology. They were commenting on the rapid expansion of RNA therapeutics in modern research and clinical development, driven in part by interest in RNA COVID-19 vaccines in the ongoing SARS-CoV-2 pandemic.
Traditionally, pharmaceutical drug development has been dominated by so-called small molecule drugs (defined as any organic compound with low molecular weight), which still have numerous applications across medicine. However, advances in biotechnology and molecular biology have since enabled researchers to design macromolecular agents ranging from monoclonal antibodies and recombinant proteins to oligonucleotides and genes/gene fragments as drug candidates. As a result, ‘biologics’ have been established as key players in the therapeutic toolbox available today. As of early 2020, biologics comprised seven of the top 10 best-selling drugs globally.
In addition, nucleic-acid-based drug design has emerged – and is currently enjoying rapid expansion. While clinical development of RNA therapeutics has traditionally been hindered by challenges such as efficiency and immunogenicity, the recent success of the mRNA COVID-19 vaccines and the approval of several RNA-based drugs has lent substantial momentum to the field. Using the CAS Content Collection™ – the largest human-curated collection of published scientific knowledge – we examine the application of RNA in modern medicine.
Read the related CAS Insights Report: RNA-Derived Medicines: A review of the research trends and developments"
Advantages and challenges of RNA therapeutics
Targeting the "undruggable"
One great advantage of RNA therapy is that RNA drugs can be used to target ‘undruggable’ molecules, which are otherwise hard or impossible to target using small molecule-based drugs. Only around one-fifth of proteins can be targeted by commonly used drugs, including small molecules and antibodies, and it is not possible to target noncoding RNAs using traditional small molecules or monoclonal antibodies (which bind to active-site pockets of protein receptors or enzymes, thus requiring translation to have occurred).
Ease of synthesis
RNA products can have major manufacturing advantages over proteins in terms of the simplicity, cost-effectiveness, and speed of the manufacturing processes made particularly relevant in recent RNA vaccine development. Nucleic-acid-based strategies can also circumvent the requirement for complex synthesis processes such as post-translational modifications by utilizing the cellular machinery of the mammalian cell.
In addition, the sequence of the RNA can be rapidly adjusted, delivering custom molecules for different targets. This greatly expedites the development process, as has clearly been the case with the rapid development of the COVID-19 RNA vaccines.
Safety and side effects
Because they enter the nucleus, DNA drugs raise safety concerns due to potential integration into the host genome. Aside from CRISPR-Cas system RNAs that edit the genome, RNAs do not alter the genomic material and carry no risk of genomic integration.
However, RNA therapeutics can have specificity issues that risk side effects, and their susceptibility to degradation can result in poor pharmacodynamics, complicating their use. Some of these issues can be mitigated by chemically modifying the RNA, a topic on which research has focused.
Delivery
RNA therapeutics tend to have a large size compared with small molecule therapeutics and have a high electric charge, making their intracellular delivery in their native forms challenging.
Research trends in RNA therapeutics
Since 1995, there has been a steady increase in the number of journals and patents containing information on RNA therapeutics – with a peak in patients around 2001 (possibly related to the first human clinical trial using dendritic cells transfected with mRNA encoding tumor antigens) and a spike in journal numbers in 2020 (most likely resulting from interest in the COVID-19 mRNA vaccines) (Figure 1).

RNA research has gradually become more diversified as new types of RNA are being discovered, particularly in the areas of siRNA, miRNA, lncRNA, and CRISPR (Figure 2). The rates of increase of publication volumes of circRNA, exosome RNA, lncRNA, and CRISPR are significantly faster than the rest. Notably, CRISPR technology accounts for 20% of the overall RNA-related patent applications in 2020. This coincides with an accelerating number of approvals for CRISPR-based therapies to enter clinical trials.
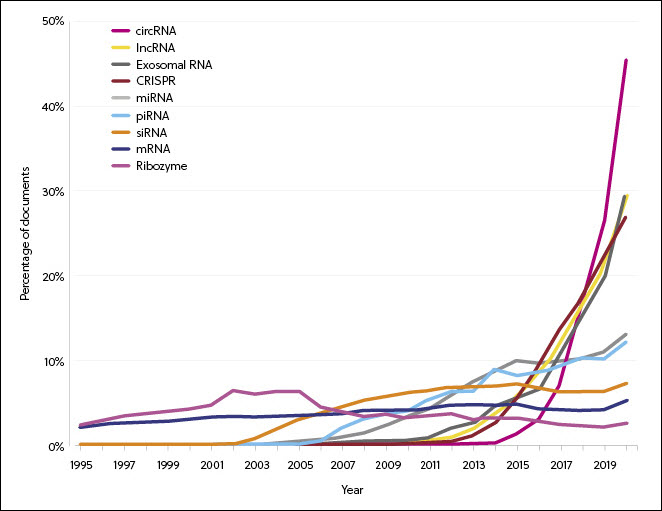
Interfering with the functionality of newly discovered RNAs is considered a promising therapeutic tool to overcome the weaknesses of traditional therapeutic approaches (Table 1).
Which therapy areas are being targeted by RNA therapeutics?
Infectious diseases and cancer show the largest growth and the greatest number of therapeutics in research phases (Figures 3 and 4). The COVID-19 pandemic has escalated RNA medicines for infectious disease in both research phases and the number of approved therapeutics (Figure 4), bringing the first approved mRNA therapeutics to market.

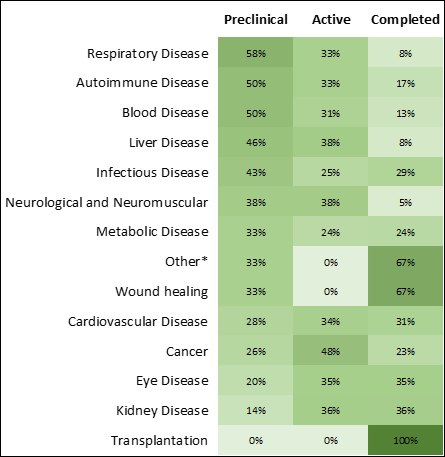
Solving challenges in RNA therapeutics
RNA chemical modification can be used to protect RNA from degradation and improve target specificity, lowering the risk of side effects due to off-target effects. In addition to chemical modification, delivery vehicles comprising nanomaterials can be used to protect the RNA from degradation and aid the transport of the therapeutic to its desired target.
According to the data in the CAS Content Collection, the use of RNA modification began to take off in 1995 and is associated with smaller sequence lengths (Figure 5). The predominance of modified 18-27-nucleotide RNAs reflects the use of this sequence length in particular forms of RNA (siRNAs and ASOs). Examination of modifications in FDA-approved RNA medicines confirms the correlation between the type of RNA and its modifications.

RNA base modifications
Non-canonical nucleotides with modifications that interfere with the formation of hydrogen bonds can thermally destabilize the formation of a duplex with the target and thus improve target specificity by limiting off-target binding. In addition, modification can improve the performance of therapeutic RNA. The use of the modified base N1-methylpseudouridine in therapeutic mRNAs, such as in COVID-19 mRNA vaccines, improves translation and lowers cytotoxic side effects and immune response to the mRNA. Both Pfizer’s Comirnaty and Moderna’s Spikevax mRNA vaccines also use 7-methylguanosine caps linked by a 5’ triphosphate to the 5’ end of the mRNA, duplicating the mRNA caps found in nature that prevent degradation of the 5’ end of the mRNA
Modifications on ribose
Modifications at the 2’ position of the ribose in RNA can increase stability and reduce off-target effects. The most common modifications at the 2’ position include 2’-O-methyl, 2’-fluoro, 2’-O-methoxyethyl (MOE), and 2’-amine.
Backbone modifications
Modifications at the phosphate group in the sugar-phosphate backbone can improve RNA delivery by neutralizing the negative charge that can interfere with transport across membranes and confer increased resistance to nucleases, thereby extending their tissue elimination half-lives. One of the most widely used backbone modifications is phosphorothioate.
RNA nanocarrier-related research
While biological barriers such as immunogenicity and nuclease stability are usually addressed by modifying the chemical structure of the RNA, additional delivery systems are necessary to overcome other barriers in the body. Encapsulation of RNA into nanoparticles is a successful way to protect and deliver RNA. Currently, there are nearly 7,000 scientific publications in the CAS Content Collection related to the RNA delivery systems. RNA carrier-related studies are dominated by the lipid nanoparticles, closely followed by the polymeric nanocarriers (Figure 6).
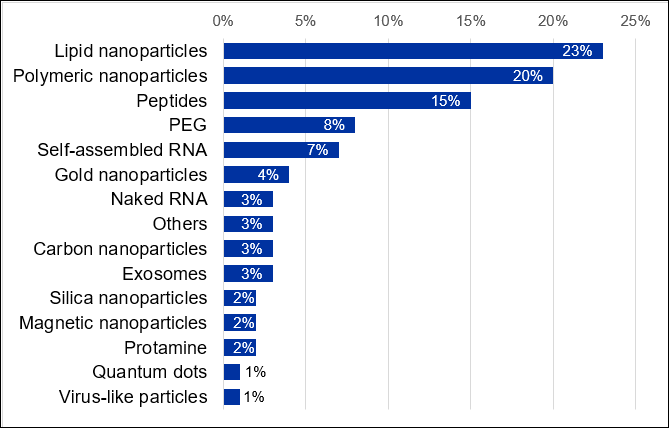
Conclusions
RNA therapeutics represent a rapidly-expanding category of drugs that are expected to change the standard of care for many diseases. They have several advantages compared with traditional medications based on small molecules and biological molecules, being cost-effective, relatively simple to manufacture, and capable of targeting previously ‘undruggable’ sites. The classical challenges experienced with their stability, delivery, and off-site effects can be eliminated or reduced via chemical modifications and RNA nanocarriers. A search in the CAS Content Collection has highlighted infectious diseases such as COVID-19 and cancer as key therapeutic areas for RNAs and shown that rates of increase of publication volumes of circRNA, exosome RNA, lncRNA, and CRISPR are particularly high – with a notable current explosion in CRISPR-related research.
Hear from the experts
To gain additional insights, view the recent ACS webinar to see what today’s leaders in RNA therapeutics are most excited by. This panel discussion features experts from diverse research backgrounds, including:
- Dr. John P. Cooke, Medical Director of the Center for RNA Therapeutics
- Dr. Robert DeLong, Associate Professor of the Nanotechnology Innovation Center at Kansas State University
- Dr. Barb Ambrose, Senior Information Scientist at CAS
- Dr. Ramana Doppalapudi, Vice President of Chemistry at Avidity Biosciences
- Moderated by Dr. Gilles Georges, Vice President and Chief Scientific Officer at CAS