
MicroRNA (miRNA/miR) are short, 20-22 nucleotide non-coding RNAs that play a critical role in gene regulation by interacting with target messenger RNA (mRNA) molecules. It is estimated that microRNAs can modulate up to 60% of protein-coding genes in the human genome at the translational level. They participate in several physiological processes, such as differentiation, proliferation, apoptosis, and development, and their dysregulation has been related to various pathological disorders.
The importance of microRNA was highlighted by the 2024 Nobel Prize in Physiology or Medicine, awarded to Victor Ambros and Gary Ruvkun “for the discovery of microRNA and its role in post-transcriptional gene regulation.” These scientists first published their discovery of microRNA in 1993, and by 2000, Ruvkun’s research group had identified let-7, a microRNA encoded by the let-7 gene, which is highly conserved across various animal species. Since then, there has been significant interest in developing microRNA therapeutics.
Issues with toxicity and effective delivery of these nucleic acids have remained a challenge. Fewer than 20 microRNA therapeutics have entered clinical trials, none of which are in phase III. Approximately half of the initiated microRNA therapeutics trials had to be suspended or terminated due to microRNA toxicity and other severe side effects.
However, new research is making progress. Advances in nanoscale drug delivery systems are addressing efficacy and toxicity issues, and microRNA therapeutics may be more widely leveraged as delivery methods continue to improve.
Biogenesis of microRNA and mechanism of action
MicroRNA can be found as independent transcriptional units or embedded within introns or exons of other genes. There are two pathways of microRNA biogenesis: the canonical pathway and the non-canonical pathway (see Figure 1).
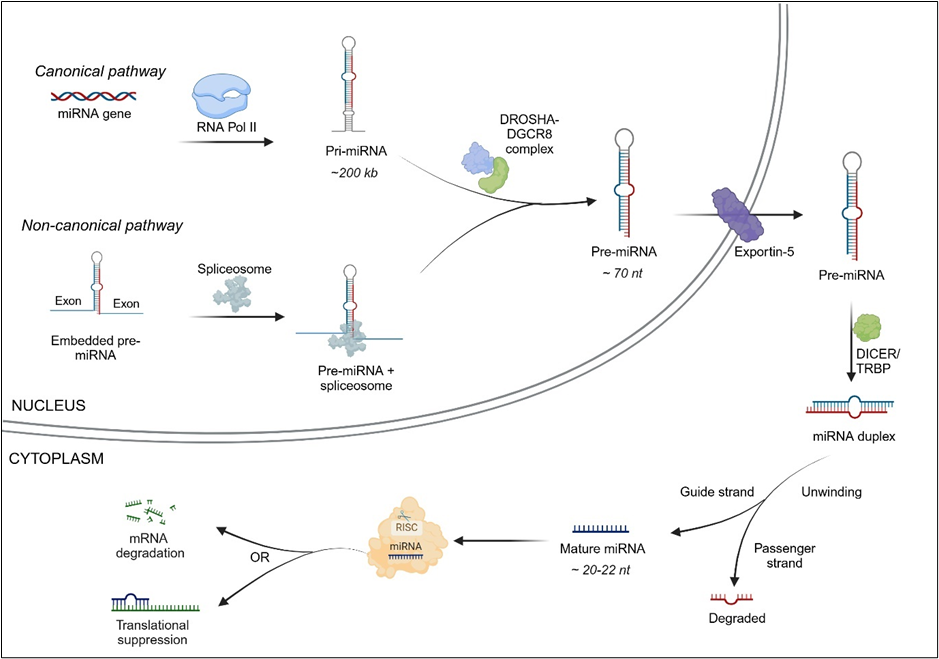
In the canonical pathway, microRNAs are transcribed from microRNA genes as long primary microRNA transcripts (pri-miRNAs) by RNA polymerase II (occasionally RNA polymerase III). Pri-miRNAs can be several kilobases long, with 5’ and 3’ flanking sequences, and can contain the stem-loops of several mature microRNAs. Pri-miRNAs are processed by the nuclear protein DGCR8 (DiGeorge syndrome critical region 8) and the enzyme DROSHA into hairpin-shaped structures called pre-miRNA transcripts.
In the non-canonical pathway, microRNA precursors located within mRNA introns are spliced out, bypassing the DGCR8/DROSHA step. Pre-miRNAs are then exported to the cytoplasm with the help of exportin-5. Once in the cytoplasm, the RNase enzyme DICER acts on the pre-miRNA hairpin loop and cleaves it to produce a mature microRNA duplex. One strand of the microRNA duplex (the guide strand) associates itself with Argonaut (AGO) protein in the RNA-induced silencing complex (RISC), while the other strand (passenger strand) is degraded. There is typically a preference for which strand is incorporated in RISC based on factors such as thermodynamic stability. Finally, the target mRNA is either degraded or undergoes translational suppression.
Improved delivery methods bring breakthroughs closer
Achieving effective delivery of microRNA therapeutics to target cells is a complex challenge owing to their instability and susceptibility to degradation. Current methodologies include viral systems (adeno-associated virus, adenovirus, and lentiviral vectors) and non-viral systems (lipid-based nanoparticles, polymer nanoparticles, inorganic nanoparticles, extracellular vesicles, and peptides).
Virus-mediated microRNA delivery has been proven effective, but this method also shows high immunogenicity. Non-viral vectors, conversely, show relative stability and low immunogenicity. As a result, researchers are exploring numerous types of non-viral nanocarriers to deliver microRNA effectively to cells.
Lipid-based nanoparticles (LNPs) are the most commonly used carriers because they protect the microRNA and have good biocompatibility. LNPs have been recently developed from ionizable cationic lipids for more efficient delivery of the microRNA. LNPs can also include “helper lipids” like cholesterol or dioleoylphosphatidylethanolamine (DOPE) to improve stability and reduce toxicity. Incorporating polyethylene glycol (PEG) has been shown to reduce nanoparticles’ aggregation and increase circulation time.
Emerging breakthroughs are also occurring with polymer-based carriers, including poly (lactic-co-glycolic acid) (PLGA). Researchers developed a microRNA-210 inhibition strategy with antisense γ peptide nucleic acids (γPNAs) with improved RNA-binding efficacy and encapsulated them in PLGA nanoparticles. PLGA has received FDA approval as a delivery vehicle and is in phase II trials for small interfering RNA (siRNA) therapies.
Inorganic nanoparticles such as gold and silica also show potential as microRNA carriers, but ongoing toxicity concerns, particularly with gold, may limit their clinical usage.
Different strategies of using microRNAs as therapeutics
We examined the CAS Content CollectionTM, the largest human-curated repository of scientific information, to better understand the research landscape of microRNA.There was a notable increase in publications relating to microRNA in the last few years, although journal publications are now decreasing slightly. However, patent publications continue to increase, suggesting sustained commercial interest in this area (see Figure 2).
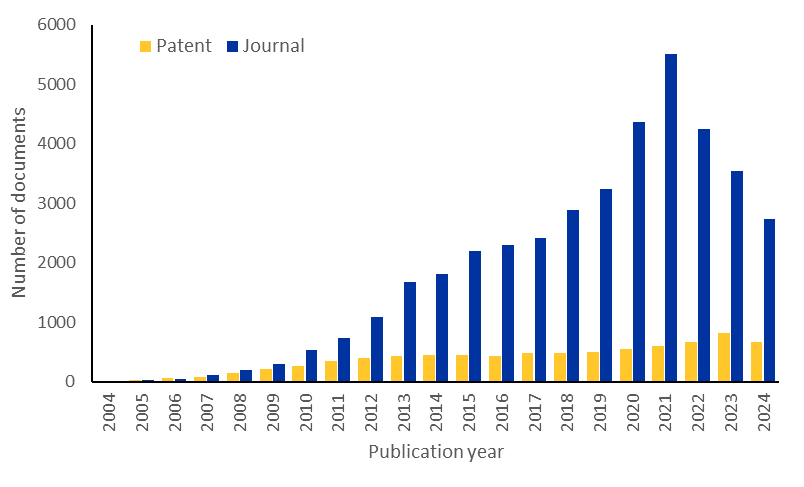
The goal of microRNA therapeutics is to alter and ideally restore abnormal or pathological microRNA expression patterns. There are currently two approaches to achieve this — microRNA stimulators or enhancers and microRNA inhibitors. The first group mainly includes microRNA mimics, while the second group comprises antiMIR oligonucleotides (AMOs), microRNA masks, sponges, and decoys. As seen in Figure 3, most publications pertain to microRNA inhibitors (67.7%).AMOs are the most common among these publications.

These various strategies work as follows:
- microRNA mimic: Synthetic double-stranded small RNA molecules that match the corresponding microRNA sequence, mimicking the action of naturally synthesized microRNA. The aim is to functionally replenish the lost or decreased microRNA expression in pathological conditions.
- antiMIRs/AMOs/anatagomiRs: Synthetic single-stranded small RNA molecules based on first-generation antisense oligonucleotides, which may have chemical modifications (e.g., antiMIRs with a 2-O-methoxyethyl modification are also called antagomiRs). These functionally bind to microRNAs with complementary sequences and prevent their interaction with target mRNA, eventually increasing their expression.
- microRNA sponges: Artificial microRNA containing tandem repeats of oligonucleotide sequences with microRNA binding sites (MBSs) for a mature microRNA of interest. They sequester the target endogenous microRNAs, leaving the MBS on target mRNAs free, thus leading to their upregulation. CircmiRs are circular microRNA sponges with the same mechanism of action but are more stable.
- microRNA decoys: A variation of microRNA sponges characterized by stem and loop structures (loop contains MBSs).
- microRNA masks: Single-stranded oligonucleotides with 2ʹ-O-methoxyethyl modification (or other chemical modification). They are perfectly complementary to the MBSs in the 3’UTR of the target mRNA, hindering the microRNA-mRNA interaction and increasing mRNA expression levels.
Exploring the potential of microRNA as therapeutics
MicroRNA could address a variety of diseases due to its range of functions in gene expression. According to our analysis of the CAS Content Collection, cancer is the most prominent therapeutic area researched (67.5%), followed by cardiovascular disorders (10.2%), infections (4.5%), diabetes (4.4%), and neurodegenerative disorders (4.3%) (see Figure 4).

Cancer
Oncogenic miRNAs (oncomiRs) influence cancer development substantially by participating in many signaling pathways, including the suppression of tumor-suppressor genes and the activation of oncogenes. The miR-34 family (miR-34a, miR-34b, miR-34c), which are under the control of TP53 tumor suppressor gene regulation, downregulate several genes, like Cyclin D1, E2, CDK4, CDK6, BCl2, and Myc, leading to cell cycle arrest and apoptosis and restricting tumor growth. The MiR-17-92 cluster, commonly referred to as “oncomir-1,” targets and inhibits tumor suppressors like PTEN, p21, and E2F, leading to increased cell proliferation, survival, and angiogenesis. MiR-21 mainly targets PTEN, PDCD4, and TIMP3 and activates survival-supportive pathways like PI3K/Akt and MAPK, further promoting tumor growth, invasion, and metastasis. MicroRNAs also play a critical role in modulating drug resistance of cancer cells apart from regulating tumor suppressors and oncogenes.
Cardiovascular diseases
Recent profiling studies have identified many microRNAs that are differentially expressed in cardiovascular diseases (CVD) in a spatiotemporal manner, pointing to a new approach to regulating conditions like myocardial infarction, atherosclerosis, heart failure, stroke, and hypertension, among others. Examples include miRNA-29 in cardiac fibrosis, miRNA-21 and miR-155 in cardiomyopathy, and miRNA-34 in myocardial infarction. MiR-21 and miR-29 also play crucial roles in extracellular matrix deposition during pathological cardiac remodeling. Dicer, an enzyme required for the maturation of all microRNAs, shows aberrant expression during heart failure. Functional studies have demonstrated that cardiac-specific knock-out of Dicer leads to progressive cardiomyopathy, heart failure, and death in mice. The available data suggests collectively that Dicer/microRNAs play critical roles in normal cardiac functions as well as under pathological conditions and are being explored as therapeutic targets in CVD.
Infections
MicroRNAs are being explored in the host-pathogen interactions of more than 50 infections (bacterial, viral, fungal, and parasitic). There are multiple ways microRNAs can help combat infectious diseases. They regulate specific proteins involved in innate and adaptive immune pathways, helping to fine-tune the immune response to infections. They can be used to target pathogen genes and inhibit their replication. For example, treatments like RG-101 and miravirsen (inhibitors of miR-122) target host microRNAs to fight hepatitis C infection. MicroRNAs are also being investigated to create attenuated vaccines by incorporating microRNA response elements into viral vaccine genomes. Some pathogens, such as Ebola, produce their own microRNAs, which can be targeted to reduce their pathogenicity and improve treatment outcomes.
Diabetes
Emerging evidence suggests that microRNAs play a key role in beta-cell functions (survival, proliferation, differentiation, and insulin secretion) and in modulating insulin resistance or sensitivity. They can influence inflammatory responses, which are typically heightened in diabetic patients. By lowering inflammation, microRNAs can assist in managing complications associated with diabetes. Several pre-clinical trials and in-vitro studies aiming to find microRNA-based therapeutics for diabetes have shown promising results.
Neurodegenerative diseases
MicroRNAs can regulate the expression of genes involved in neurodegenerative diseases, reduce neuroinflammation, and protect neurons from apoptosis. Examples include miR-124 and miR-132, which support neuronal health and function, and the miR-29 and miR-34 families that target genes associated with amyloid-beta production in Alzheimer’s disease. MicroRNAs can additionally promote neurogenesis, which is crucial for brain repair, with miR-9 and miR-124 known to play important roles in neurogenesis. Dysregulated microRNAs can lead to neurological deterioration and the development of neurodegenerative diseases. Therefore, modulating these microRNAs provides a novel direction for treating these conditions.
We plotted the most commonly occurring microRNAs in the literature dataset and visualized their distribution across the top five therapeutic areas as part of our analysis (see Figure 5).
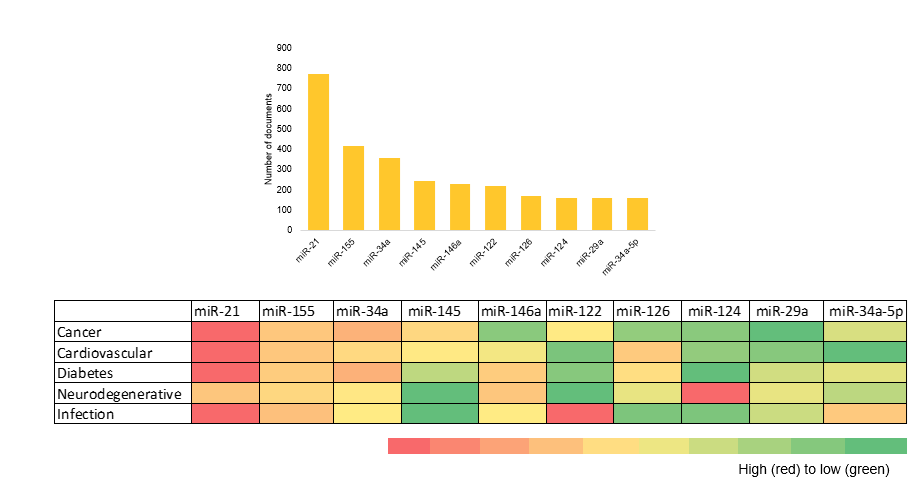
We also conducted a co-occurrence analysis to determine which types of microRNA therapies are most closely associated with the five leading conditions in the literature (see Figure 6).

Outlook for microRNA therapeutics
We have seen promising new advances in microRNA therapies. However, persistent challenges remain to overcome before these treatments can be used in clinical practice. Clinical trials must take place to confirm the safety and efficacy of different microRNA strategies and delivery methods. Undesired immune response, non-specific tissue accumulation, and endosomal degradation are other significant challenges in developing microRNA-based therapeutics.
Recent research demonstrates that breakthroughs are occurring, and the future is bright for these therapies. Advanced technologies like CRISPR/Cas to target microRNA genes and artificial intelligence-based models to predict microRNA targets demonstrate exciting opportunities for developing microRNA therapeutics.
MicroRNA therapies could soon transform patient care for numerous diseases through continued innovation.