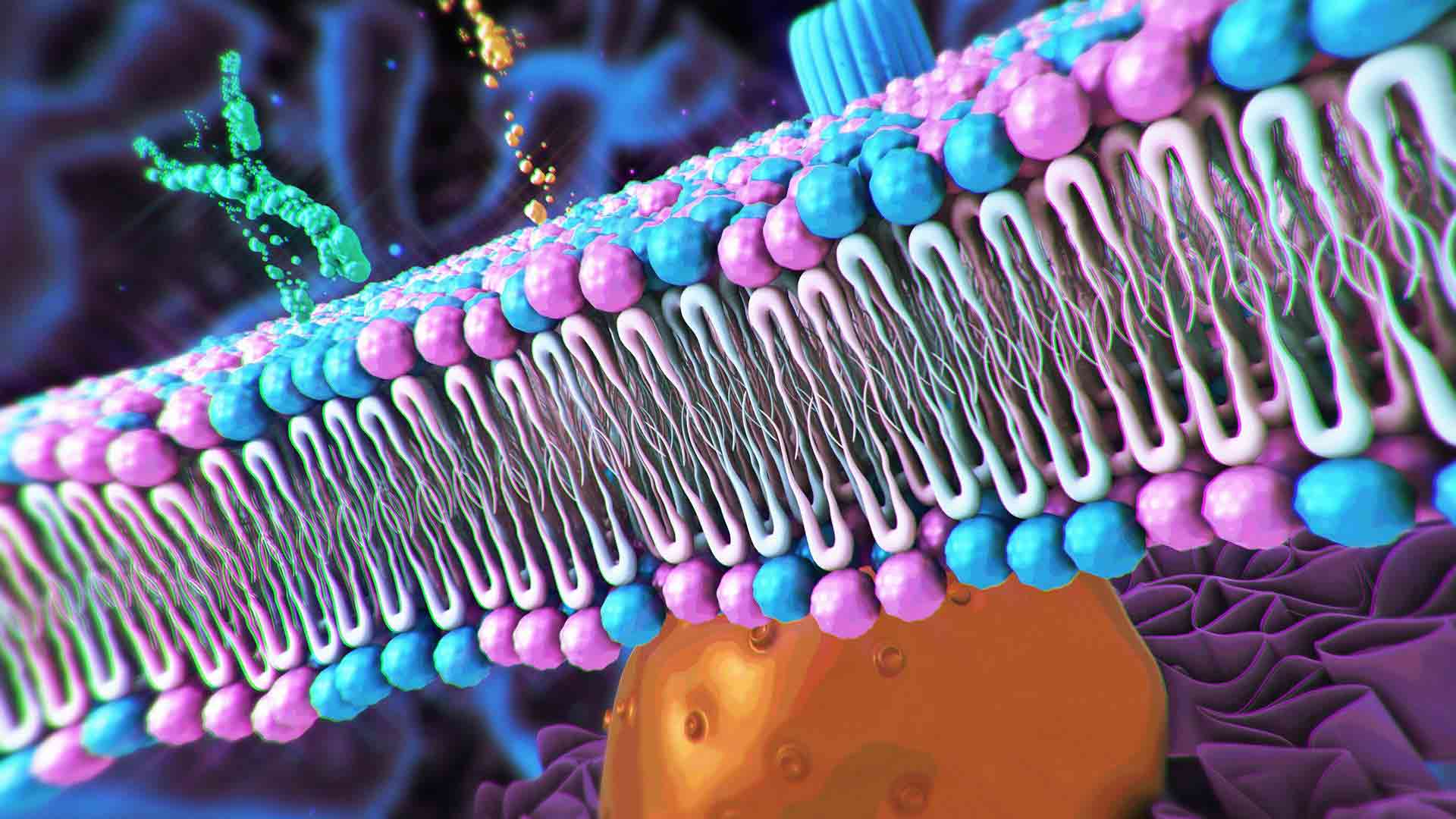
Lipids have become indispensable to modern drug delivery methods thanks to their biocompatibility, versatility, and ability to encapsulate different therapeutic agents. As essential components of biological membranes, lipids offer unique physicochemical properties that address critical challenges in drug delivery, including poor solubility, low bioavailability, rapid degradation, and off-target effects. The field has witnessed remarkable innovations in recent years, from simple liposomes to advanced systems such as solid lipid nanoparticles (SLNs) and stimuli-responsive lipids.
Where is research heading in this growing field of study? What types of lipids may be the next breakthrough in drug delivery systems (DDS)? We delved deep into the CAS Content CollectionTM, the largest human-curated repository of scientific information, to fully understand the landscape of lipid DDS research and identify key trends and developments. The result is our new report, Lipid-Based Drug Delivery Systems: Unlock the Future of Drug Delivery, which shares cutting-edge research advancing the field of drug delivery via lipid-based systems.
Here, we provide a snapshot of what we uncovered—the designs of the most commonly cited lipid-based DDS in the CAS Content Collection. This analysis demonstrates which lipids are the workhorses of DDS research and where discoveries are pushing boundaries for better precision medicine.
{{lipid-report="/new-components"}}
What are lipid DDS?
Lipids, the cornerstone of biological membranes, exhibit a remarkable affinity for both hydrophilic and hydrophobic moieties, endowing them with unparalleled biocompatibility and bioavailability. This inherent flexibility has spurred their widespread adoption across many drug delivery modalities, ranging from simple liposomal encapsulation to intricate lipid-based nanoparticles. Indeed, one of the most notable advantages of lipid-based DDS is their ability to encapsulate a broad spectrum of compounds, including small molecule drugs, nucleic acids, and peptides.
The lipid bilayer structure also protects the encapsulated cargo, shielding it from enzymatic degradation and premature clearance while facilitating controlled release kinetics at the target site. Because of these properties, lipid-based DDS are used for vaccines—including mRNA vaccines like those for COVID-19—cancer treatments, gene therapy, and the delivery of medications to challenging systems in the body. For example, some lipid-based DDS are used to treat neurological disorders because they can cross the blood-brain barrier and enhance drug retention in the eye, making them useful for ophthalmic conditions.
We analyzed the CAS Content Collection and found steady, consistent growth in lipid DDS-related publications over the last 20 years (see Figure 1). Since 2010, lipid DDS publications have surpassed overall lipid-related ones and have consistently grown faster. The patent-to-journal ratio is also higher for lipid DDS documents than the same ratio for overall lipid-related documents, indicating greater commercial interest.

Figure 1: (A) Publications in lipid-based DDS and related research over the last two decades (2003-2024). Data includes journal (blue) and patent (yellow) publications. (B) Comparison of the publication yearly growth (clustered columns) and the patents-journal ratio (lines) for the overall lipid-related publications (orange) vs. lipid DDS-related ones (blue). *Indicates partial data for 2024 and includes data only for the months January to May 2024. Source: CAS Content Collection.
With continued innovations in lipid DDS, such as the recent success of mRNA vaccines, it is understandable that excitement in this field is growing. Let’s explore some of the most prominent types of these DDS, based on our analysis of over 200,000 indexed scientific documents for our new report:
Lipid DDS designs for target precision and drug efficiency
Lipid DDS comprise a diverse array of forms, ranging from liposomes to micelles to exosomes. Our full-text report also reviews virus-like particles and stimuli-responsive systems of liposomes, including echogenic liposomes. Here, we discuss a few of the most notable types of lipid DDS:
Liposomes
Liposomes are the most prominent type of lipid DDS in the literature, and it is easy to see why—they laid the foundation for the development of nanocarriers in drug delivery, becoming the first nanomedicine delivery platform to successfully progress from concept to clinical application with a number of approved pharmaceutical preparations.
Liposomes consist of one or several lipid bilayers, ranging in size between 20 and approximately 1000 nm. They can encapsulate both hydrophilic and hydrophobic drugs. Hydrophilic drugs can be enclosed in the aqueous interior of liposomes, while hydrophobic drugs can be entrapped in the hydrocarbon chain region of the lipid bilayer, making liposomes a resourceful and versatile drug delivery platform.
Nanosized liposomes have recently been engineered with two discrete compartments, one within another, termed concentrisomes or liposomes-in-liposomes. By controlling the composition of each bilayer and specifying the identity of encapsulated cargo within each compartment, it has become possible to imbue each bilayer with different stimuli-responsive properties, enabling these particles to achieve multi-stage release of two payloads at specific defined points in time.
Due to their versatility, liposomes have been studied as carriers for many types of drugs, such as small molecules, proteins, and genes (see Figure 2). They have been used in numerous clinical trials to deliver anticancer, anti-inflammatory, antibiotic, and antifungal drugs.
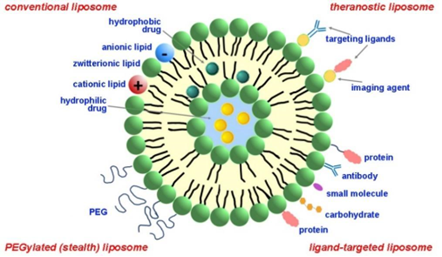
Figure 2: Schematic depicting various types of pharmaceutical liposomes. Source: CAS.
Solid lipid nanoparticles (SLNs) and nanostructured lipid carriers (NLCs)
All liposomes can be considered a type of lipid nanoparticle, but solid lipid nanoparticles (SLNs) and nanostructured lipid carriers (NLCs) are the next generation of lipid nanocarriers. They were developed to address problems with liposome production, including organic solvents, low encapsulation efficiency, and difficulties scaling up production.
Although conventional liposomes comprise liquid crystalline lipid bilayers, SLNs are composed of solid lipids, and NLCs are mixtures of solid and liquid crystalline lipids (see Figure 3). The typical sizes of SLNs and NLCs are between 40 and approximately 1000 nm. These advanced LNPs exhibit enhanced physical stabilities, higher loading capacities, and higher bioavailabilities of their cargoes. They are easily manufactured at large scales without using organic solvents and tolerate sterilization better than other LNPs. Moreover, the limited molecular mobility in the solid state allows for more precise control of drug release from SLNs and NLCs.
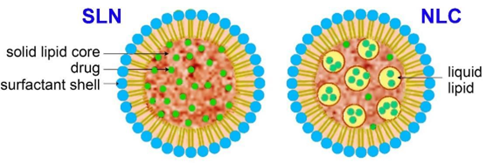
Figure 3: Schematic presentation of solid lipid nanoparticles (SLNs) and nanostructured lipid carriers (NLCs). Source: CAS.
The latest successful use of SLNs is as the delivery vehicle in the two recently approved COVID-19 mRNA vaccines by Pfizer/BioNTech and Moderna, which were developed with unparalleled speed and have shown notable effectiveness in disease prevention. These advances demonstrate the potential of SLNs and NLCs to drive new DDS breakthroughs.
Lipoplexes
Nucleic acids hold promise as gene therapy agents. However, their delivery to cells presents significant challenges. The negative electric charge and hydrophilicity of nucleic acids inhibit passive diffusion across plasma membranes. Additionally, the association of nucleic acids with serum proteins, uptake by phagocytes, and degradation by endogenous nucleases interferes with successful delivery. Therefore, nucleic acids require delivery vectors for more efficient uptake.
Enter lipoplexes—cationic LNPs forming stable complexes between synthetic cationic lipids and anionic nucleic acids. Lipoplexes deliver genetic material into cells effectively because they protect the nucleic acids from degradation, facilitate cellular uptake, and promote intracellular trafficking to the nucleus or cytoplasm. Their structure can range from spherical particles to more complex multilamellar structures, depending on the lipid-to-DNA/RNA ratio and lipid composition (see Figure 4).

Figure 4: Schematic presentation of (A) lamellar, (B) hexagonal, and (C) cubic lipoplexes. Source: CAS.
Association with positively charged lipids stabilizes nucleic acids and enhances their resistance to nuclease degradation, enabling delivery to target cells. Nucleic acids enter the cells by adsorption of the lipoplexes to the cell surface, followed by their endocytosis and release into the cell.
Adsorption of the lipid carriers to and fusion with cell membrane are driven electrostatically because cell membranes normally bear a negative charge, and the lipoplex lipids bear a positive charge. Their electrostatic attraction causes membrane fusion and endocytosis.
The release of nucleic acids from their complexes with cationic lipids is necessary for successful delivery once inside the cell. The anionic lipids of the cellular membrane likely assist in releasing nucleic acids from lipoplexes by neutralizing the charge of the cationic lipid carriers, eliminating the electrostatic interactions between the lipid carriers and the cargo (nucleic acids). Blending anionic lipids with the cationic lipids also disrupts the nanoparticle architecture, leading to the formation of non-lamellar structures, which are beneficial for nucleic acid release.
Non-lamellar lipid nanoparticles
Technologies relating to the use of non-lamellar lipid phases in drug delivery and the use of inverted cubic and hexagonal liquid crystalline phases in controlled-release formulations for the delivery of inhaled drugs were first published in the 1980s. These later emerged as a promising class of advanced carriers for the next generation of nanomedicines. Their unique structural properties and tunable characteristics make them promising candidates for developing advanced DDS with improved efficacy and specificity.
Cubosomes, highly stable nanoparticles formed from lipid cubic phases and stabilized by polymer-based outer coronas, were developed more recently as lipid pharmaceutical nanocarriers (see Figure 5).
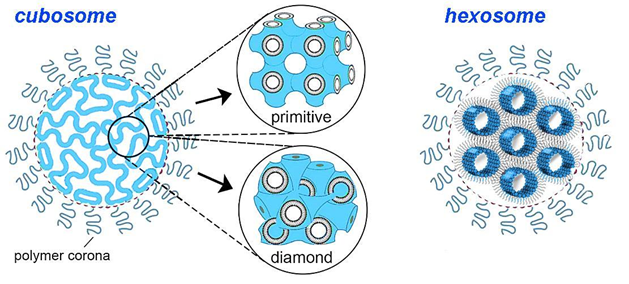
Figure 5: Schematic presentation: cubosome nanoparticles (left) comprising lipid in a bicontinuous bilayer cubic phase (either primitive (Im3m) or diamond (Pn3m) type); 3 hexosome nanoparticle (right) comprising lipid in an inverse hexagonal phase. Source: CAS.
Self-assembled cubosomes have drawn much attention as active drug delivery vehicles since their discovery. They possess a bicontinuous cubic structure with interconnected water channels, providing a large surface area for drug loading. They are suitable for encapsulating hydrophobic, hydrophilic, and amphiphilic drugs and can be surface-modified for targeted delivery.
Additional advantages of non-lamellar LNPs include high drug dispersal due to the cubic phase’s structure, a relatively simple manufacturing process, biodegradability, and controlled release of bioactive agents.
Future directions for lipid-based DDS innovations
The demand for personalized DDS will continue to grow as the pharmaceutical industry moves towards more precision medicine approaches. Developing more sophisticated LNP systems, including SLNs, NLCs, and other liposomes, will play a pivotal role in targeted drug delivery. These DDS could result in breakthroughs in cancer treatment and gene therapies. As we have seen with COVID-19 vaccines, other mRNA vaccines could be brought to market faster with lipid DDS technology.
Explore our full-text report, Lipid-Based Drug Delivery Systems: Unlock the Future of Drug Delivery, to learn more about the current state of lipid DDS research and emerging advancements. The future of lipid-based DDS is bright and holds promising developments for patients worldwide.